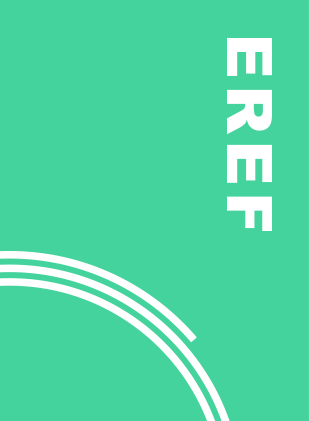
Description
It has been estimated that the manufacturing of nanoparticles (NPs) will increase from 1,000 to 58,000 tonnes yearly from 2011 to 2020 (Royal Society and Royal Academy of Engineering, 2004). As NPs continue to be incorporated into consumer products, the introduction of these materials to landfills and the environment is inevitable. To fully understand the environmental implications of NPs use, knowledge regarding their mobility, bioavailability, and ecotoxicity is important (Farré et al., 2009).
This research sought to understand the fate of NPs within landfills by examining the interactions between NPs and landfill leachate components. The primary focus of this study was to evaluate the effect of Zinc Oxide (ZnO), Titanium Dioxide (TiO2), and silver NPs (Ag) on biological landfill processes, solids aggregation, and chemical speciation of Zinc (Zn), Titanium (Ti), and Silver (Ag) in landfill leachate following the addition of crystalline, nano-sized ZnO, TiO2, and Ag. This research (1) observed the effects of coated NPs on leachate five-day biochemical oxygen demand (BOD5) and biochemical methane potential (BMP), (2) examined effects of solids aggregation on the fate of NPs, (3) characterized the NPs before and after addition to leachates, (4) quantified the concentration of metals associated with the added NPs by size fractions, and (5) modeled the chemical speciation of Zn, Ti, and Ag in landfill leachate using Visual MINTEQ. The NPs studied were chosen for their common use in personal care products which accounted for just over 55% of the inventory of NP-containing consumer products available in 2010 (Nanotech-Project, 2011).
Key findings include the following:
- It was observed that humic acids (HA) greatly increased the dispersibility of the coated ZnO NPs, which can be attributed to over-coating of NP surface by HA through hydrophobic-hydrophobic interaction. Fourier Transform Infra-Red (FT-IR) spectroscopy and fluorescence characterization data confirmed that HA was adsorbed onto coated NPs, increasing their wettability. Dispersion of TiO2 and Ag NPs was also observed but needs to be investigated further.
- High Resolution Transmission Electron Microscopy images suggested that ZnO NPs were embedded in leachate matrices after addition to leachate samples. TiO2 was observed embedded in purchased HA.
- Dynamic Light Scattering and Atomic Force Microscopy data revealed that dispersed ZnO NPs tended to agglomerate in leachate. Fractionation studies also showed that ZnO as well as Ag NPs agglomerated in leachate.
- Zn and Ag were found at concentrations above background in some leachate size fractions between 1 and 1500 nm which can be attributed to leachate components interacting with the NP coatings resulting in NP dispersion, dissolution, agglomeration, and/or dissociation. This fate accounted for less than 1% of the mass of NP added.
- An increase in the dissolved concentration of all tested NPs was observed in some leachates, which supports dissociation of these NPs, however this fate accounted for less than 1% of the mass of NP added.
- ZnO, Ag, and TiO2 NPs did not affect biological processes when added to middle-aged and mature leachate as measured by Biochemical Oxygen Demand and Biological Methane Production tests.
- MINTEQ models showed that dissolved Zn, Ag, and Ti in leachate were associated with HA, NH3/Cl, and hydroxide, respectively. Since these components are frequently found in leachate at relatively high concentrations, toxicity would be reduced for NPs containing these metals if the NPs dissociate.
NP characterization, leachate size fractionation, BOD5 and BMP tests, and chemical speciation modeling provided insight into the mobility of NPs in landfills and the absence of inhibitory effects on landfill processes. Aggregation of NPs may prevent movement through traditional containment systems (i.e. geomembrane liners) due to the increased particle size. However, the increased dispersion suggests that NPs may be transported out of the landfill in the leachate. Nevertheless, it was found that biological treatment of leachate should be unaffected by the presence of NPs. The toxicity of the metals associated with the added NPs should be limited by the affinity of dissolved Zn for dissolved organic matter (e.g. HA), Ti for hydroxide, and Ag for NH3 and Cl-. The inconsistent observation of NPs in different leachate fractions are due to the heterogeneity of landfill leachate and the utilization of different NP coatings; therefore it will be challenging to predict mobility of other NPs in other landfills.
Overall the understanding of the fate of coated NPs and their behavior in landfill leachate will directly benefit landfill operations and leachate management. Research data can aide regulatory agencies in addressing potential nanotoxicological issues and develop a knowledge base to enhance the understanding of the fate and transport of NPs in landfills. Such understanding will ensure that these materials are properly managed from cradle to grave, while protecting human health and the environment.
It is recommended that regulations be implemented to ensure safe disposal and manufacturing of NPs. Unfortunately, due to the lack of consumer product composition, particularly pertaining to the NP concentration, coating, and size, it will be difficult to implement regulations that would be applicable to all NPs. In order to develop regulations, it will be important to determine a key parameter that could predict mobility in the environment based solely on the characteristics that can be provided by a manufacturer (hydrophilic/hydrophobic, coating composition, and size). Overall the development of such regulations will be challenging without more detailed knowledge of the fate and transport of NPs in MSW landfills, as well as the environment.
$0.00